High resolution spectroscopy using optical frequency combs
We are developing a new scheme for high resolution spectroscopy of infrared absorption using a laser called optical frequency comb. Details are as follows.
High resolution spectroscopy
First of all, high resolution spectroscopy. There are quite a lot of studies that light is applied to a substance (anything is fine), and that reaction is seen. At this time, a method of seeing the reaction change when changing the wavelength (frequency) of light is generally called "spectroscopy". The resolution is how finely the frequency of this light can be distinguished. For example, suppose the case in which difference of 1 GHz of light frequency at wavelength 500 nm (green, frequency is 6 × 1014 Hz) can be distinguished (that is, the difference between 6.000 000 × 1014 Hz and 6.000 001 × 1014 Hz). Similarly, suppose the case in which the difference of 1 MHz can be distinguished (that is, the difference between 6.000 000 000 × 1014 Hz and 6.000 000 001 × 1014 Hz). Then the latter is three orders of magnitude higher (or better) in resolution.
Why high resolution spectroscopy is important
High-resolution spectroscopy is in general very difficult, and then it is not a good idea to make anything high resolution. The extent of high resolution varies depending on what you are observing for spectroscopy. We observe absorption of simple gas molecules in the infrared wavelength range (from about 800 nm to about 2000 nm). In this wavelength range, for example, there is absorption such as methane molecule.
This absorption occurs only at certain light frequencies. Which frequency of light is absorbed depends on the distribution of the energy level of methane. For example, if you use a database called HITRAN, you can see at which frequency (wavelength) and how strong the absorption occurs. The figure below shows the position where the absorption spectrum of methane molecule is located using HITRAN. The horizontal axis is the unit of "wave number" (cm-1), which is the inversel of the wavelength of light. 1 cm-1 corresponds to 30 GHz. The vertical axis is the absorption intensity.
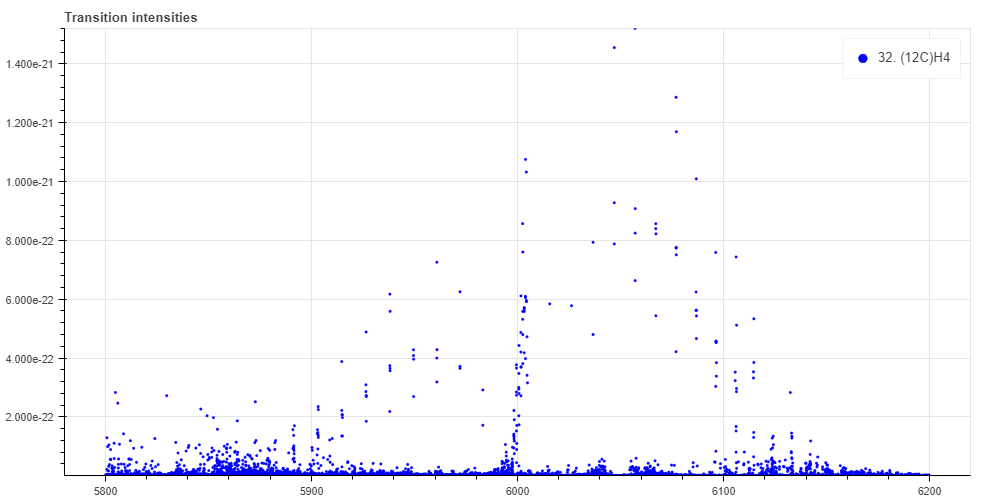
Each one of these transitions is broadened by about 0.2 GHz. The reason for this spectral broadenenig is that molecules are thermally moving around and cause a Doppler effect, so that for each molecule the frequency of light seems to be a slightly different frequency.
In fact, the broadening of the absorption spectral line due to the Doppler effect can be canceled by some sophisticated scheme. Then, the absorption spectral line becomes thinner. Accurately measuring this transition frequency makes it possible to accurately measure the energy level structure of methane. Such measurements can be used, for example, to verify the theory of quantum mechanics, time dependence of physical constants, physical model verification, etc. It also helps to improve the accuracy of atomic clocks. It may be possible to measure the structure of the nucleus in the future as the accuracy further improves. At present, the energy necessary for usual elementary particle experiment is becoming too high to be handled by human beings. Even in such experiments,we believe that it can be made cheaper with high resolution spectroscopy.
Another important application of high resolution spectroscopy is remote sensing. Remote sensing is a technology to detect the concentration of methane gas in the atmosphere using light, for example. This is exactly the application of spectroscopy. In particular, methane is one of the major greenhouse gases, so it is an important object to detect by remote sensing. With high resolution spectroscopy, the shape of the spectral line can be measured accurately. As stated before, the shape of the spectral line depends on the thermal motion (temperature) of the gas. In other words, measuring the shape of the spectral line accurately is equivalent to the gas temperature measurement. Besides, the shape of the spectral line depends on the pressure, and the center frequency of the spectral line corresponds to the direction in which the gas molecule is moving. In addition, when other absorption spectra of methane is measured at the same time, the abundance ratio of isotopes can be measured. By measuring the spectral line at high resolution, it is expected that not only the concentration but also other physical quantities can be measured at the same time.
We are studying high-resolution spectroscopy using an optical frequency comb.
Optical frequency combs
Optical frequency comb generators are essentially ultrashort pulse lasers. Optical frequency distribution of this laser light consists of many narrow spectral lines at regular intervals as shown below. This interval coincides with the repetition frequency of the pulse, and the bandwidth of the spectrum is about the inversel of the pulse width. Since this shape looks like comb teeth, this device is called an "optical frequency comb generator". Since the teeth of the comb are spaced at regular intervals, it can be used as an optical frequency measure, and hence the optical frequency can be easily measured.
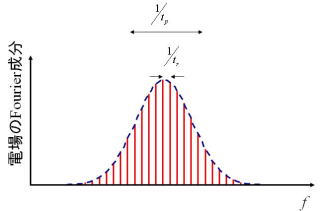
Direct comb spectroscopy
Since the optical frequency comb is like a laser oscillating at the same time in a very wide wavelength range, broadband spectroscopy using this as a spectroscopic light source is an active research area (direct comb spectroscopy). A recently developed and powerful scheme is to use two optical frequency combs called "dual comb spectroscopy". In our laboratory, we use direct comb spectroscopy using one optical frequency comb and one continuous-wave (cw) laser. With this scheme, although the spectral bandwidth is narrower than that of dual comb spectroscopy, the spectral data can be obtained very rapidly. Taking advantage of this feature, we are trying to apply to high resolution spectroscopy.
High resolution direct comb spectroscopy
The resolution of comb direct spectroscopy is determined by the repetition
rate of the optical frequency comb. For high-resolution spectroscopy, optical
frequency combs with a slow repetition rate is required. However, it is
difficult to make such optical frequency combs because of technical problems.
Therefore, we are developing a scheme to reduce the repetition rate of
the optical frequency comb substantially (1 / integer) by "electro-optical
modulation".
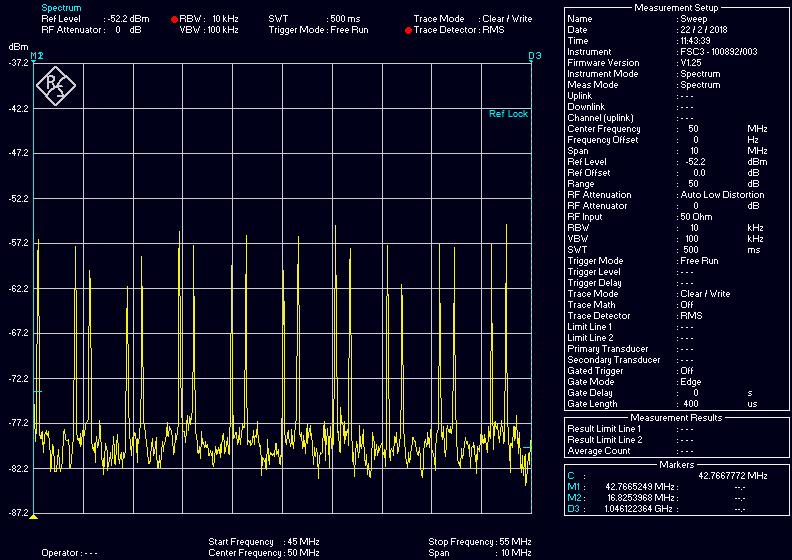